The aviation industry is a major contributor to global greenhouse gas emissions, driven by its dependence on conventional fossil fuels. As the demand for air travel rises, the urgency for sustainable alternatives, such as Sustainable Aviation Fuels (SAF), becomes increasingly clear. SAFs have the potential to reduce carbon emissions by up to 80% compared to traditional jet fuels, positioning them as a powerful solution for decarbonizing the aviation sector (Han et al., 2025). Understanding the combustion properties of sustainable aviation fuels (SAFs) and the significance of kinetic modeling in combustion processes are essential for optimizing their application in aviation.
Importance of Kinetic Modeling in Combustion Processes
Kinetic modeling serves as a vital tool in enhancing our understanding of fuel combustion behavior, particularly for sustainable aviation fuels (SAFs). By developing mathematical models that accurately depict the chemical reactions involved in combustion, we can make valuable predictions about key parameters such as ignition delay times, flame speeds, and emissions profiles. This approach not only advances our knowledge but also contributes to the optimization of fuel performance and environmental impact.
Kinetic models are essential for:
- Predicting Combustion Behavior: Kinetic models predict the behavior of different fuels during combustion, which is essential for achieving efficient and clean combustion in aircraft engines (Wang et al., 2025).
- Optimizing Fuel Formulations: By understanding reaction mechanisms and kinetics, researchers can optimize fuel formulations to improve performance and minimize emissions. This is especially important for sustainable aviation fuels (SAFs), which may differ in chemical composition from conventional fuels (Rijal et al., 2025).
- Streamlining Certification Processes: The airworthiness certification of sustainable aviation fuels (SAFs) requires a comprehensive understanding of their physicochemical properties and combustion characteristics. Kinetic modeling can facilitate this process by providing insights into the combustion chemistry of SAFs, promoting their large-scale adoption (Wang et al., 2025).
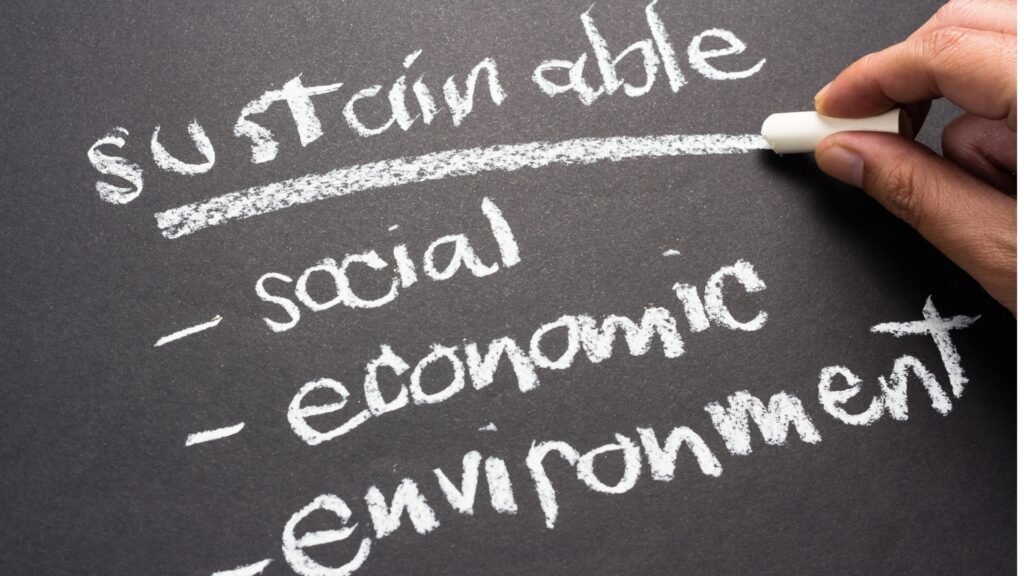
Relevance of Combustion Properties to Sustainable Aviation Fuels (SAF)
The combustion properties of sustainable aviation fuels (SAFs) are critical for their performance in aviation applications.
Key properties include:
- Ignition Delay Time (IDT): IDT is a vital parameter that influences engine performance and emissions. SAFs must have suitable IDT characteristics to ensure compatibility with existing aircraft engines (Wang et al., 2025).
- Flame Speed: The flame speed of a fuel affects the stability and efficiency of combustion. SAFs that exhibit appropriate flame speeds can enhance combustion efficiency and reduce the formation of harmful emissions (Wang et al., 2025).
- Emissions Characteristics: SAFs are engineered to reduce emissions of pollutants like particulate matter and nitrogen oxides. Understanding the combustion properties of SAFs enables the design of combustion systems that effectively lower these emissions (Xu, Fan, et al., 2025).
- Thermal Stability and Energy Density: The thermal stability and energy density of sustainable aviation fuels (SAFs) are crucial for their performance in aviation. These fuels must maintain stability under the varying temperature and pressure conditions encountered during flight (Rijal et al., 2025).
Kinetic Modeling in Combustion Processes
Kinetic modeling is a mathematical method used to describe the rates of chemical reactions and the mechanisms through which they occur. In the context of combustion, kinetic modeling is essential for predicting how fuels behave during combustion processes. This understanding is crucial for optimizing engine performance and reducing emissions. Kinetic models provide insights into how different fuels react under various conditions, enabling the design of more efficient combustion systems (Wang et al., 2025).
The main goal of kinetic modeling is to offer insights into the intricate interactions among fuel components, reaction intermediates, and products. By simulating these interactions, researchers can predict ignition delay times, flame speeds, and emissions profiles. These predictions are essential for the development of sustainable aviation fuels (SAF) and other alternative fuels. (Ramos-Fernandez et al., 2025).
Modeling Approaches
Kinetic modeling can be categorized into several approaches, each with its advantages and limitations:
- Detailed Chemical Kinetics:
- This method uses detailed reaction mechanisms that consider all possible reactions and species involved in the combustion process. Comprehensive kinetic models can provide highly accurate predictions of combustion behavior.
- Advantages: High accuracy and the ability to capture complex reaction pathways.
- Limitations: This process is computationally intensive and may need a significant amount of experimental data for validation (Wang et al., 2025).
- Reduced Mechanisms:
- Reduced mechanisms simplify detailed kinetic models by concentrating on significant reactions and species. This method is particularly beneficial for large-scale simulations where computational efficiency is essential.
- Advantages: Faster computations and reduced complexity while still capturing essential combustion characteristics.
- Limitations: Potential loss of accuracy and the risk of omitting important reactions that could influence combustion behavior (Wang et al., 2025).
- Surrogate Models:
- Surrogate models use simplified representations of fuel compositions to predict combustion behavior. These models are often based on a limited number of representative compounds that mimic the properties of more complex fuels.
- Advantages: Easier to implement and can be tailored to specific fuel types.
- Limitations: May not fully capture the nuances of complex fuel compositions (Wang et al., 2025).
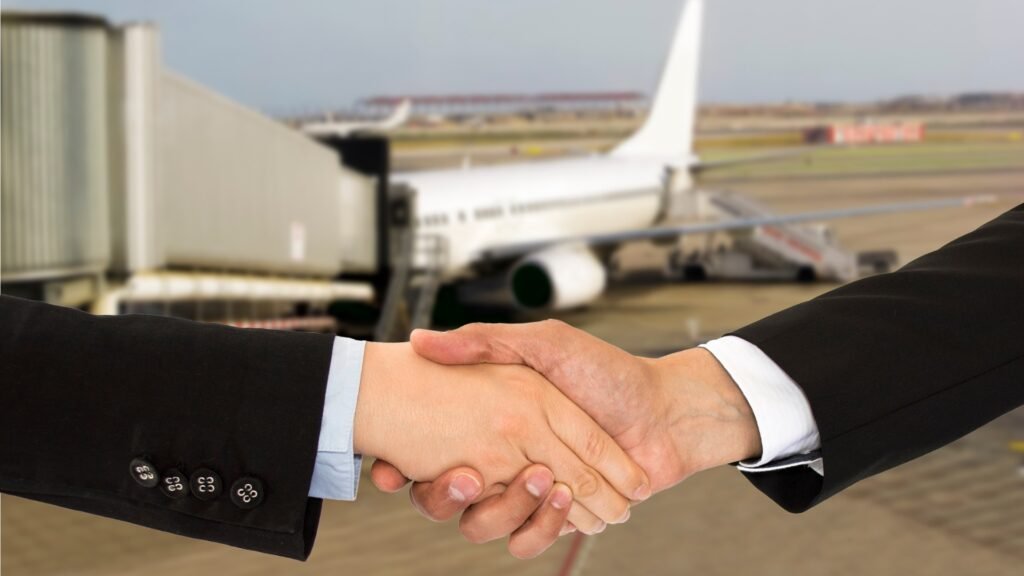
Key Parameters in Kinetic Modeling
Several key parameters are critical in kinetic modeling, influencing the accuracy and reliability of predictions:
- Reaction Rates:
- Reaction rates are fundamental to kinetic modeling, as they determine how quickly reactants are converted into products. These rates are influenced by factors such as temperature, pressure, and the presence of catalysts (Wang et al., 2025).
- Activation Energy:
- Activation energy is the minimum energy required for a reaction to occur. It plays a crucial role in determining the temperature dependence of reaction rates. Higher activation energies typically result in slower reaction rates at lower temperatures (Wang et al., 2025).
- Temperature Dependence:
- The Arrhenius equation describes how reaction rates change with temperature, highlighting the exponential relationship between temperature and reaction rate. Understanding this dependence is essential for accurately modeling combustion processes (Wang et al., 2025).
- Influence of Fuel Composition:
- The composition of the fuel significantly affects reaction kinetics. Different fuel components can lead to variations in ignition delay times, flame speeds, and emissions profiles. For instance, the presence of aromatics or specific hydrocarbons can alter the combustion characteristics of a fuel (Wang et al., 2025).
Combustion Properties of Sustainable Aviation Fuels
1. Physical and Chemical Properties of Fuels
The combustion performance of fuels is significantly influenced by their physical and chemical properties. Key properties include:
- Density: The density of a fuel affects its energy content and the amount of fuel required for combustion. Higher density fuels typically contain more energy per unit volume, which can enhance engine performance (Xu, Shi, et al., 2025).
- Viscosity: Viscosity impacts the atomization of fuel during injection into the combustion chamber. Fuels with lower viscosity tend to atomize better, leading to more efficient combustion and reduced emissions (Xu, Shi, et al., 2025).
- Flash Point: The flash point is the lowest temperature at which a fuel can vaporize to form an ignitable mixture in air. Fuels with higher flash points are generally safer to handle and store, but may require higher temperatures for effective combustion (Xu, Shi, et al., 2025).
These properties collectively influence the combustion efficiency, stability, and emissions profile of the fuel. For instance, sustainable aviation fuels (SAFs) like Hydroprocessed Esters and Fatty Acids (HEFA) exhibit physical and chemical properties that are comparable to conventional jet fuels, allowing for seamless integration into existing aviation infrastructure (Han et al., 2025).
2. Ignition Characteristics
Ignition Delay Times: Ignition delay time (IDT) is a critical parameter in evaluating fuel performance in engines. It represents the time interval between the start of fuel injection and the onset of combustion. Shorter IDTs are generally favorable as they lead to more efficient combustion and improved engine response (Wang et al., 2025).
- Significance in Engine Performance: IDT affects engine performance metrics such as power output and fuel efficiency. Fuels with longer IDTs may lead to incomplete combustion, resulting in increased emissions and reduced engine efficiency (Wang et al., 2025).
- Comparison Between Conventional Fuels and SAF: Studies have shown that SAFs, particularly those produced via the HEFA process, can exhibit shorter IDTs compared to traditional jet fuels. This characteristic can enhance the combustion process, leading to lower emissions of unburned hydrocarbons and particulate matter (Xu, Shi, et al., 2025).
3. Emissions Characteristics
The emissions produced during combustion are a critical aspect of evaluating fuel performance and environmental impact. Key emissions include:
- CO2 Emissions: SAFs can reduce lifecycle CO2 emissions by up to 80% compared to conventional jet fuels, primarily due to the biogenic carbon cycle, where the CO2 released during combustion is offset by the CO2 absorbed during the growth of feedstocks (Han et al., 2025).
- NOx Emissions: Nitrogen oxides (NOx) are significant contributors to air pollution and can lead to the formation of smog and acid rain. The combustion of SAFs has been shown to produce lower NOx emissions compared to traditional fuels, attributed to their lower aromatic content (Xu, Fan, et al., 2025).
- Particulate Matter: SAFs, particularly those that are free of aromatics, can significantly reduce particulate matter emissions. Studies indicate that the use of SAFs can lead to a reduction in particle numbers by an order of magnitude, which is crucial for improving air quality around airports (Xu, Fan, et al., 2025).
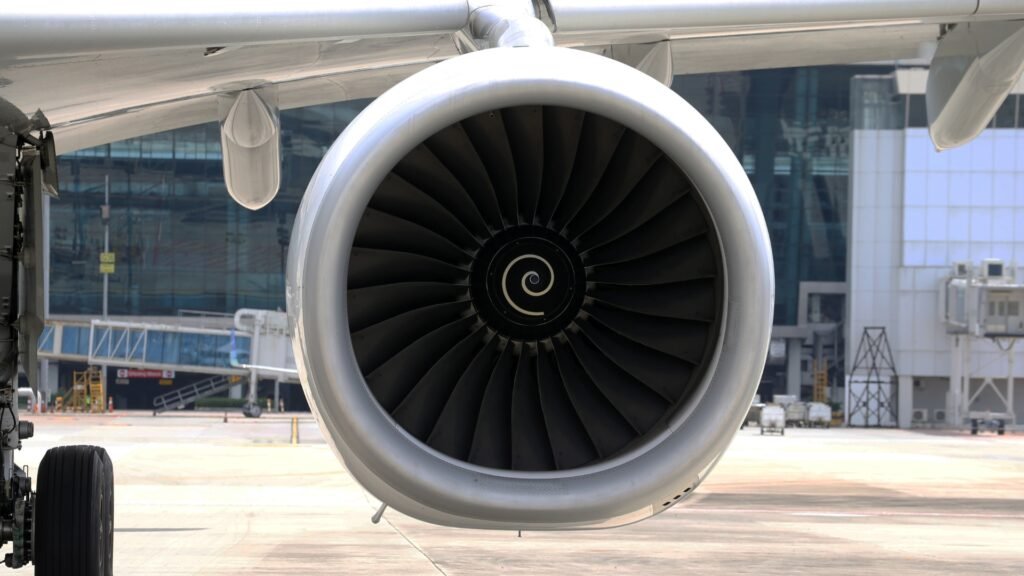
Experimental Methods in Combustion Properties Measurement
1. Techniques for Measuring Combustion Properties
The measurement of combustion properties is critical for understanding fuel performance and optimizing combustion processes. Various experimental setups and techniques are employed to obtain accurate data on ignition delay times, emissions, and other combustion characteristics.
1.1 Experimental Setups
- Shock Tube: The shock tube is a widely used experimental apparatus for studying ignition kinetics and combustion properties. It allows for the generation of high-temperature and high-pressure conditions that simulate engine environments. The shock wave generated in the tube compresses the gas mixture, leading to rapid heating and ignition. This setup is particularly useful for measuring ignition delay times (IDTs) of fuels under controlled conditions (Wang et al., 2025).
- Combustion Chamber: Combustion chambers are designed to facilitate the study of fuel combustion under various conditions, including different pressures and temperatures. These chambers can be equipped with diagnostic tools such as laser diagnostics, which provide real-time measurements of species concentrations and temperature profiles during combustion. The use of optical techniques allows for detailed analysis of flame structure and emissions (Wang et al., 2025).
1.2 Methods for Measuring Ignition Delay Times and Emissions
- Ignition Delay Time Measurement: IDTs can be measured using various methods, including the shock tube technique and rapid compression machines. In the shock tube, the time from the start of fuel injection to the onset of combustion is recorded, providing a direct measurement of IDT. Rapid compression machines allow for the simulation of engine-like conditions, enabling the measurement of IDTs at varying pressures and temperatures (Wang et al., 2025).
- Emissions Measurement: Emissions from combustion processes are typically analyzed using Fourier Transform Infrared (FTIR) spectroscopy, gas chromatography (GC), and flame ionization detectors (FID). FTIR can measure multiple gaseous species simultaneously, while GC is used for detailed analysis of volatile organic compounds (VOCs) and particulate matter (PM). These methods provide insights into the emissions profile of different fuels, including CO2, CO, NOx, and unburned hydrocarbons (Rohkamp et al., 2025).
2. Data Analysis
The analysis of experimental data is crucial for validating kinetic models and understanding combustion behavior. Various approaches are employed to ensure the reliability and accuracy of the results obtained from experimental setups.
2.1 Approaches for Analyzing Experimental Data
- Statistical Analysis: Statistical methods are used to analyze the variability and reliability of experimental data. This includes calculating mean values, standard deviations, and confidence intervals to assess the precision of measurements. For instance, the relative standard deviation can be calculated to quantify the consistency of IDT measurements across multiple trials (Rohkamp et al., 2025).
- Kinetic Model Validation: The data obtained from experiments are often compared with predictions from kinetic models to validate their accuracy. This involves using the measured IDTs and emissions data to refine and adjust the parameters of the kinetic models. Sensitivity analysis can also be performed to identify which parameters most significantly affect the model predictions, thereby guiding further experimental investigations (Wang et al., 2025).
- Life Cycle Assessment (LCA): In addition to combustion properties, LCA can be employed to evaluate the environmental impacts of different fuels. This comprehensive approach considers all stages of fuel production and use, providing insights into the overall sustainability of various fuel pathways (Greene et al., 2025).
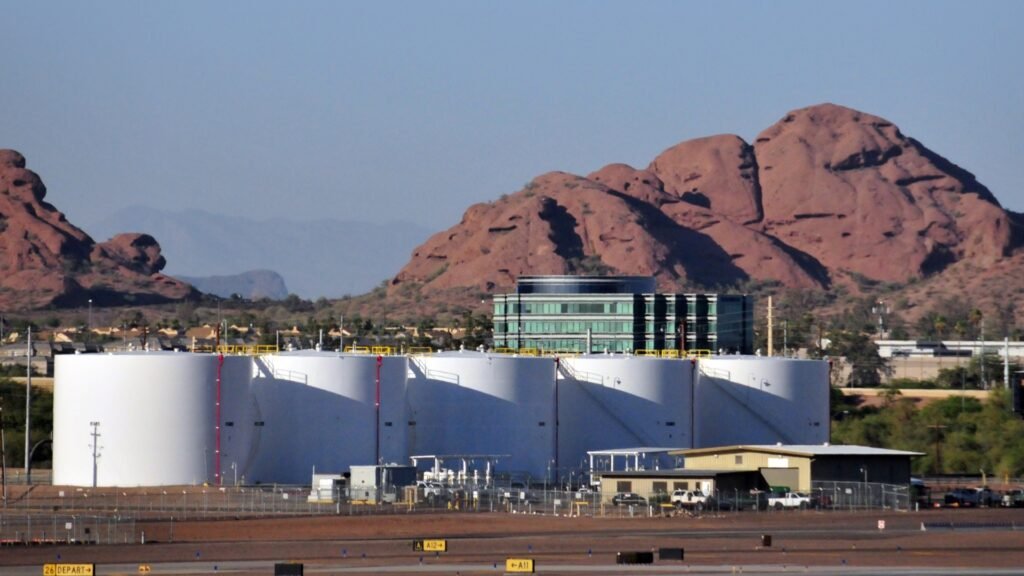
Case Studies on Kinetic Models and Combustion Properties of Sustainable Aviation Fuels (SAF)
1. Application of Kinetic Models to SAF
Kinetic modeling plays a crucial role in understanding the combustion behavior of Sustainable Aviation Fuels (SAFs), particularly in optimizing their performance and emissions profiles. Several studies have focused on different production pathways for SAF, including Hydroprocessed Esters and Fatty Acids (HEFA) and Fischer-Tropsch (FT) synthesis.
- HEFA Kinetic Modeling: Research has demonstrated that HEFA fuels, derived from the hydroprocessing of vegetable oils, exhibit distinct combustion characteristics compared to conventional jet fuels. For instance, a study utilizing a shock tube facility to measure ignition delay times (IDTs) of HEFA blends with traditional RP-3 jet fuel found that the kinetic mechanisms developed could accurately predict the combustion behavior of these blends. The study highlighted the importance of understanding the physicochemical properties of HEFA to streamline the airworthiness certification process (Wang et al., 2025).
- Fischer-Tropsch Kinetic Modeling: The Fischer-Tropsch synthesis pathway is another significant method for producing SAF. Kinetic models developed for FT fuels have shown that these fuels can achieve negative greenhouse gas emissions under certain conditions, particularly when combined with carbon capture and storage (BECCS). Studies have indicated that FT pathways can produce fuels with lower emissions compared to conventional fuels, emphasizing the need for detailed kinetic mechanisms to optimize the combustion process (Yang & Yao, 2025).
2. Comparison of Combustion Properties
Numerous studies have compared the combustion properties of SAFs with those of conventional jet fuels, revealing significant differences in emissions and performance.
- Emissions Profiles: A comprehensive analysis of various SAF pathways, including HEFA and FT, indicates that SAFs can reduce carbon emissions by up to 80-95% compared to traditional fossil fuels, depending on the feedstock and production method used (Jiang & Liu, 2025); (Han et al., 2025). For example, SAF produced via the HEFA process has been shown to significantly lower particulate matter emissions due to its lower aromatic content, which is a critical factor in reducing the environmental impact of aviation (Xu, Fan, et al., 2025).
- Combustion Efficiency: Studies have also highlighted differences in combustion efficiency between SAFs and conventional fuels. SAFs, particularly those produced through the FT process, have been found to exhibit higher energy densities and improved combustion characteristics, which can lead to enhanced engine performance. The combustion of SAFs typically results in lower emissions of nitrogen oxides (NOx) and unburned hydrocarbons, contributing to better air quality around airports (Rijal et al., 2025); (Bardon et al., 2025).
- Kinetic Behavior: The kinetic behavior of SAFs, including their ignition delay times and flame speeds, has been extensively studied. Research indicates that SAFs often have shorter ignition delay times compared to conventional fuels, which can enhance combustion efficiency and reduce the formation of harmful emissions (Wang et al., 2025); (Lu et al., 2025). This is particularly relevant for the aviation industry, where optimizing fuel performance is critical for meeting stringent emissions regulations.
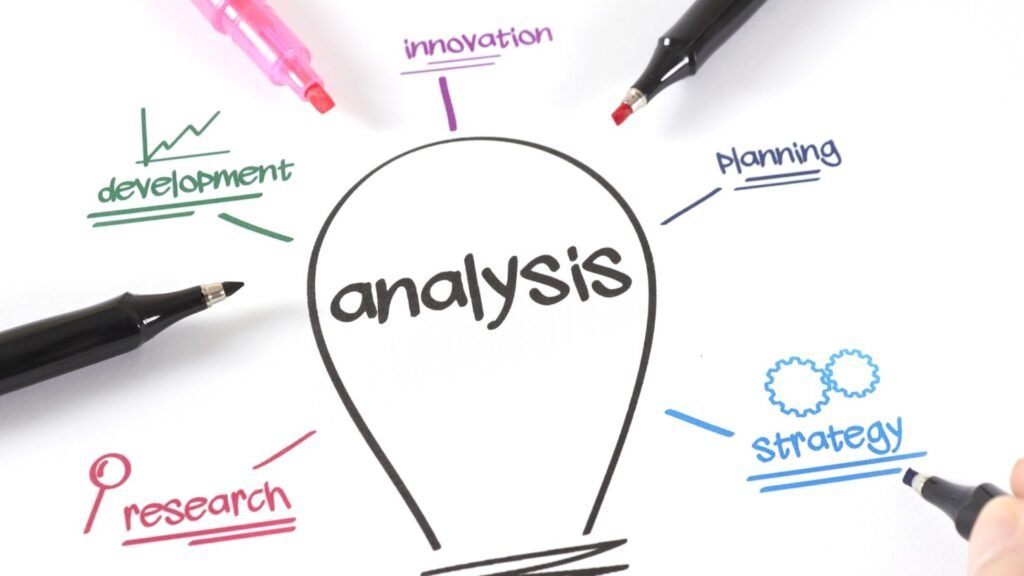
The Role of Kinetic Modeling in Understanding Combustion Properties and Its Implications for Sustainable Aviation Fuels
1. Importance of Kinetic Modeling in Understanding Combustion Properties
Kinetic modeling is a critical tool in the study of combustion processes, particularly for understanding the behavior of fuels under various conditions. It provides insights into the reaction mechanisms, ignition characteristics, and emissions profiles of different fuels, which are essential for optimizing combustion efficiency and reducing environmental impacts.
- Predictive Capability: Kinetic models enable researchers to predict how fuels will behave during combustion, including ignition delay times (IDTs) and flame propagation. For instance, studies utilizing shock tube facilities have demonstrated the effectiveness of kinetic models in accurately predicting the IDTs of sustainable aviation fuels (SAFs) and their blends with conventional fuels (Wang et al., 2025). This predictive capability is vital for the design of combustion systems that maximize efficiency and minimize emissions.
- Understanding Reaction Mechanisms: Kinetic modeling helps elucidate the complex chemical reactions that occur during combustion. By developing detailed and lumped kinetic mechanisms, researchers can identify the key species and reactions that influence combustion behavior. This understanding is crucial for improving fuel formulations and optimizing combustion chamber designs to enhance performance (Wang et al., 2025).
- Emissions Reduction: Kinetic models are instrumental in assessing the emissions profiles of different fuels. For example, SAFs have been shown to significantly reduce carbon emissions compared to traditional jet fuels, with reductions of up to 85% depending on the production pathway (Lu et al., 2025). Kinetic modeling allows for the evaluation of how different fuel compositions affect emissions, guiding the development of cleaner fuels.
2. Implications for the Development and Optimization of Sustainable Aviation Fuels
The insights gained from kinetic modeling have significant implications for the development and optimization of sustainable aviation fuels. As the aviation industry seeks to reduce its carbon footprint, SAFs are emerging as a viable alternative to conventional fossil fuels.
- Fuel Formulation: Kinetic modeling informs the formulation of SAFs by identifying the optimal blend of feedstocks and production processes. For instance, the hydroprocessed esters and fatty acids (HEFA) process is one of the most common methods for producing SAFs, and kinetic models can help optimize the reaction conditions to enhance yield and reduce costs (Han et al., 2025). Understanding the combustion properties of these fuels through kinetic modeling is essential for ensuring their compatibility with existing aviation infrastructure.
- Regulatory Compliance and Certification: The airworthiness certification of SAFs requires a thorough understanding of their physicochemical and combustion properties. Kinetic modeling aids in this process by providing the necessary data to meet regulatory standards, such as those set by ASTM International (Wang et al., 2025). This is crucial for facilitating the large-scale adoption of SAFs in commercial aviation.
- Long-Term Sustainability: Kinetic modeling also plays a role in assessing the long-term sustainability of SAFs. By evaluating the lifecycle emissions and economic viability of different production pathways, researchers can identify the most sustainable options for fuel production (Jiang & Liu, 2025). This is particularly important as the aviation industry aims to achieve net-zero emissions by 2050, necessitating a shift towards more sustainable fuel sources.
References
Bardon, P., Massol, O., & Thomas, A. (2025). Greening aviation with sustainable aviation fuels: Insights from decarbonization scenarios. Journal of Environmental Management, 374, 123943. https://doi.org/10.1016/j.jenvman.2024.123943
Greene, J. M., Quiroz, D., Limb, B. J., & Quinn, J. C. (2025). Geographically-resolved techno-economic and life cycle assessment comparing microalgae-based renewable diesel and sustainable aviation fuel in the United States. Environmental Science & Technology, 59(7), 3472. https://doi.org/10.1021/acs.est.4c06742
Han, J., Matsumoto, T., Yamada, R., & Ogino, H. (2025). Reshaping the substrate-binding pocket of acyl-ACP reductase to enhance the production of sustainable aviation fuel in Escherichia coli. Biotechnology and Bioengineering, 122(1), 211-222. https://doi.org/10.1002/bit.28863
Jiang, C., & Liu, Y. (2025). The role of sustainable aviation fuel in CORSIA: An economic analysis. Energy Economics, 143, 108238. https://doi.org/10.1016/j.eneco.2025.108238
Lu, F., Li, L., Yu, Y., Tian, G., Xiong, H., Miao, J., Wei, F., & Zhang, C. (2025). Road to carbon-neutral aviation: One-step conversion of CO2 to sustainable aviation fuels. Industrial & Engineering Chemistry Research, 64(6), 3118-3135. https://doi.org/10.1021/acs.iecr.4c04209
Ramos-Fernandez, E. V., Santos, J. L., Alsaadi, D. K., Bavykina, A., Gallo, J. M. R., & Gascon, J. (2025). Potential pathways for CO2 utilization in sustainable aviation fuel synthesis. Chemical Science (Cambridge), 16(2), 530-551. https://doi.org/10.1039/d4sc06164k
Rijal, D., Vasilyev, V., & Wang, F. (2025). Advancing sustainable aviation fuel design: Machine learning for high-energy-density liquid polycyclic hydrocarbons. Energy & Fuels, 39(6), 3243-3255. https://doi.org/10.1021/acs.energyfuels.4c06147
Rohkamp, M., Rabl, A., Bendl, J., Neukirchen, C., Saraji-Bozorgzad, M. R., Helcig, C., Hupfer, A., & Adam, T. (2025). Gaseous and particulate matter (PM) emissions from a turboshaft-engine using different blends of sustainable aviation fuel (SAF). Aerosol Science and Technology, 59(1), 111-126. https://doi.org/10.1080/02786826.2024.2417977
Wang, Q.-D., Zeng, P., Yao, Q., Liang, J., Wang, B.-Y., Huang, F., Liu, J.-G., & Xia, Z.-X. (2025). An experimental and kinetic modeling study on the ignition kinetics of a sustainable aviation fuel and its blends with a traditional RP-3 jet fuel. Fuel (Guildford), 380, 133191. https://doi.org/10.1016/j.fuel.2024.133191
Xu, Z., Fan, Y., Zheng, Y., Ding, S., Zhu, M., Li, G., Wang, M., Yu, Z., Song, Y., Chang, L., & Chen, L. (2025). Emission reduction characteristics of heavy-fuel aircraft piston engine fueled with 100% HEFA sustainable aviation fuel. Environmental Pollution (1987), 368, 125661. https://doi.org/10.1016/j.envpol.2025.125661
Xu, Z., Shi, W., Wang, M., Zhong, S., Zhou, Y., Pei, J., Shao, L., Pan, K., & Song, Y. (2025). Performance and combustion characteristics of heavy-fuel aircraft piston engines at high altitudes: Comparison between conventional fuels and HEFA sustainable aviation fuel. Sustainable Energy Technologies and Assessments, 75, 104210. https://doi.org/10.1016/j.seta.2025.104210
Yang, F., & Yao, Y. (2025). Sustainable aviation fuel pathways: Emissions, costs and uncertainty. Resources, Conservation and Recycling, 215, 108124. https://doi.org/10.1016/j.resconrec.2025.108124